AMD has introduced the Versal AI Edge XQRVE2302, a compact adaptive SoC designed for space applications. It is significantly smaller than its predecessor and incorporates enhanced AMD AI Engine (AIE) technology optimized for machine learning, supporting data types like INT4 and BFLOAT16 for tasks such as anomaly and image detection. These devices allow unlimited reprogramming during development and after deployment, even in space’s challenging radiation environment, and offer security features to prevent tampering and configuration changes. They have demonstrated radiation tolerance for missions from low-earth orbit to geosynchronous earth orbit and beyond. While modern FPGAs are preferred for space and aviation systems, rigorous testing and quality control are essential to ensure their suitability for radiation-prone environments, with traceability and change control being key factors.
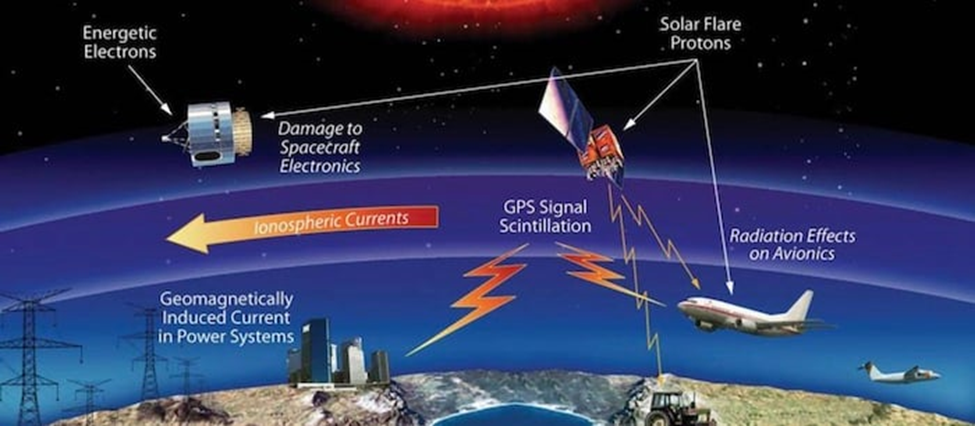
What do we think? With process nodes approaching the angstrom domain, the susceptibility of integrated circuits to radiation disturbances is greater than ever. Combine that with an increasing demand for space-worthy circuits for airplanes, satellites, and spaceships and you have a perfect storm. Clever circuit design can mitigate a lot of issues before they occur.
AMD’s compact adaptive SoC to help design radiation-hardened electronics for space environments
AMD is expanding its space-grade adaptive compute solutions with the Versal AI Edge XQRVE2302, an adaptive SoC offered for space flight.
Notably, the device is compact, measuring 23×23mm, and significantly smaller than its predecessor, the Versal AI Core XQRVC1902. It incorporates enhanced AMD AI Engine (AIE) technology, known as AIE-ML, and, says AMD, is optimized for machine learning applications, particularly ML inference, with support for data types like INT4 and BFLOAT16. That makes it suitable for tasks like anomaly and image detection.
Importantly, XQR Versal adaptive SoCs enable unlimited reprogramming during development and after deployment, even in the challenging radiation environment of space. They also offer security features to prevent tampering and unwanted configuration changes, allowing flexibility in remote sensing and communications applications for satellite operators.
The XQR Versal SoC devices have demonstrated radiation tolerance for missions from low-earth orbit to geosynchronous earth orbit and beyond. AMD says pre-production devices are available now, with flight-qualified parts expected in late 2024.
Radiation effects in electronics can be classified into two main categories: single-event effects (SEEs) and total ionizing dose (TID) effects. SEEs occur when a single sub-atomic particle interacts with a semiconductor. In terrestrial electronics, common causes of SEEs are alpha particles from impurities in semiconductors or packaging materials and neutrons from atmospheric radiation. In space, SEEs are primarily caused by highly energetic protons and heavy ions due to the absence of atmospheric shielding. These particles, concentrated by Earth’s magnetic field, are significantly more damaging than their terrestrial counterparts.
Single-event upsets (SEUs) are a specific type of SEE where a flip-flop or SRAM cell experiences a sudden current pulse due to ionization and recombination near a PN junction when a charged particle passes through the semiconductor. Neutrons, despite being electrically neutral, can also trigger SEUs when they collide with silicon atoms, generating charged particles. SEUs can flip the state of a memory element, turning a logic 1 into a logic 0 or vice versa. While SEUs might have minimal impact at the system level, they are crucial to consider in radiation-hardened electronics design for space applications.
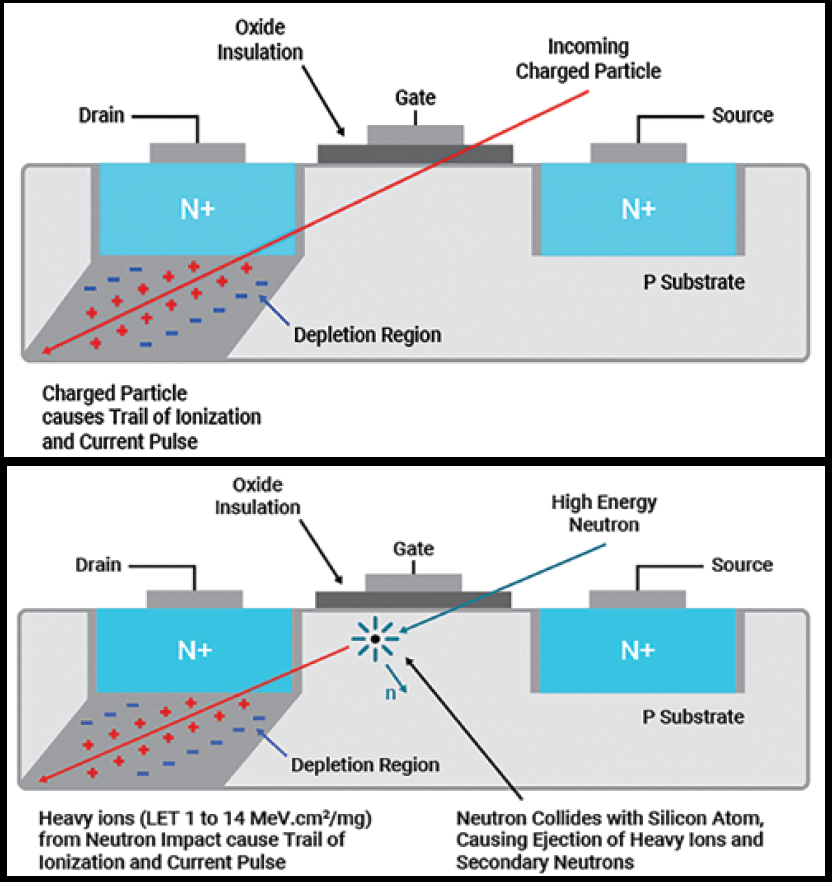
The radiation tolerance of AMD’s Versal Adaptive SoC products comes partly from intrinsic properties of manufacturing process (primarily radiation total dose effects) and from deliberate measures they took in the design of the parts. Specific design rules were adopted to mitigate single event latch-ups, and over 50 patents were filed on other radiation mitigation techniques to mitigate uncorrectable errors and to provide protection against single event upsets. These steps were taken to mitigate radiation effects in ground level and airborne applications and not just for space, although space applications obviously benefit from them. There is no shielding or coating involved in improving radiation characteristics of the Versal adaptive SoC devices.
Modern FPGAs have become the preferred choice for space and aviation systems. However, they must withstand radiation effects, even while operating at ground level. In many instances, the devices are designed to tolerate radiation in airborne and space applications. Nonetheless, thorough testing is critical before finalizing a design for such critical applications.
Maintaining traceability and change control is also crucial to ensuring that the test data collected prior to procuring flight-ready components remains pertinent to the components being sourced.
COTS (Commercial Off-The-Shelf) components are often considered for these applications, but stringent testing and quality control are necessary to ensure their suitability for use in radiation-prone environments.